Problem and Challenges:
The potential of mmWave technology (30 GHz to 300 GHz, but usually also frequencies above 10 GHz are included) for future mobile networks led to a significant investment in research in the form of H2020 projects and specifically several large 5G Public-Private Partnership (PPP) projects. It also motivated the European Commission to recommend opening up parts of the mmWave spectrum for broadband services. At the same time, enabling communications at mmWave frequencies remains challenging. The mmWave radio propagation behaviour is characterized by high path loss and penetration loss, which limits the native communication range of mmWave signals to a few meters. Highly directional beamforming antennas can mitigate the impact of path loss and increase the communication range, but this in turn requires fast and precise beam alignment which in itself is challenging in dynamic mobile environments. Moreover, mmWave signals are easily blocked by most objects, such as walls, the human body, etc. Preliminary studies on mmWave communications and early trials have thusfocused onshowing the feasibility of multi-Gbps data rates in low-density scenarios with limited dynamics such as fixed point-to-point links. Products focusing on such relatively static scenarios (e.g., fixed wireless access, WLAN 802.11ad) are already available on the market. However, these products are not designed for mobile environments and may suffer from frequent connection outages due to the inefficiency of their beamforming and interference control techniques.
Research Opportunities:
Although high directionality and penetration loss pose challenges, they are outweighed by the potential gains in data rate and reduction in latency because of the extremely small transmission delay. In addition, the range limitations and directional communication also bring important advantages, including low interference and high spatial reuse for extremely dense deployments. Furthermore, mmWave and conventional low frequency communications have highly complementary characteristics and integrating both allows to simultaneously provide high performance as well as reliability and resilience (allowing the overall network to continue to provide service in the face of outage or faults in one of the sub-networks). The vastly different network characteristics have set off a chain reaction, requiring major changes throughout the different layers of the protocol stack. These architecture and protocol design choices at the physical layer, the network layer, and the application itself are highly interdependent and need to be considered jointly. The primary goal of this ETN is to train ESRs to co-create the required mmWave algorithms and protocols together with the novel use cases mmWave technology is supposed to enable. Specifically, providing continuous and reliable connectivity in extremely dense and highly mobile scenariosis an open challenge, and understanding how to tune a mmWave network towards a specific future application with its particular mix of throughput, latency and resilience requirements is still in its infancy. This is not only important for ubiquitous mobile broadband services, but is a key requirement for the forthcoming industry 4.0, vehicle-to-everything (V2X), augmented reality (AR) and similar new applications. In addition, the large available bandwidth at mmWave frequencies enables highly accurate environment sensing and localization, adding new important network capabilities that can support such use cases. The high diversity of the requirements of these different application scenarios (in terms of latency, mobility, resilience, etc.), together with new sensing capabilities, call for adaptive and tailored network solutions rather than use case-agnostic one-size-fits-all designs. The latter is a major obstacle for the adaptation of current mobile broadband services (e.g., LTE) in manufacturing industries. Being able to adapt mmWave technology to different use cases is expected to foster a market growth in the 2019-2023 period at a CAGR of 103.7%. This is therefore an excellent time to act in order to make a significant and durable impact on the mmWave technology market (Figure 1).
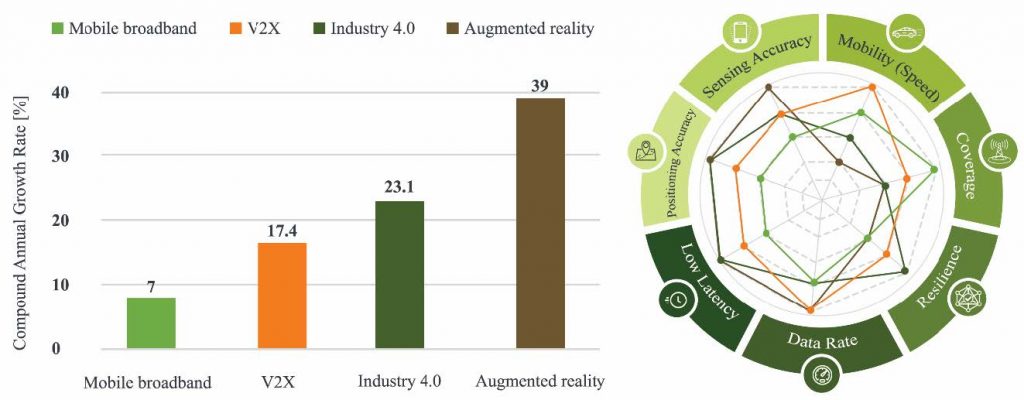
Figure 1. The bar chart shows the potential CAGR growth for the main mmWave applications and performance requirements: Besides high data rates, there are different application-dependent key requirements for sensing, positioning, mobility, coverage, resilience and latency that have to be met at the system level.
Project Objectives
1) building up a highly skilled labour force in the mmWave technology sector;
2) mitigating the impact of network dynamics and density on the performance and resilience of mmWave networks;
3) advancing mmWave technology in order to leverage it in emerging EU and international markets.
Project Aim
The project aims at technology for mmWave networking and sensing beyond 5G. This beyond 5G technology will give us even larger capacity, more diverse applications, such as very precise indoor location and radar, in addition to communication. The beyond 5G networks will however also require more antennas, and work at the so feared high mmWave frequencies (feared already for 5G).